The Quest for Understanding the Universe
In the vast landscape of scientific discovery, few fields have the potential to redefine our understanding of the universe as profoundly as particle physics. While many experiments contribute to this pursuit, some of the most groundbreaking research takes place in massive particle accelerators and computational projects dedicated to simulating fundamental interactions. As scientists continue to explore the unknown, projects such as LHC@home provide a glimpse into the collaborative efforts required to push the boundaries of physics.
The Evolution of Particle Physics
Since the early 20th century, physicists have sought to understand the fundamental building blocks of nature. The development of quantum mechanics and relativity revolutionized our perception of the microscopic and macroscopic worlds, leading to the establishment of the Standard Model of particle physics. This framework successfully describes the interactions of elementary particles but leaves several questions unanswered, particularly regarding dark matter, dark energy, and the unification of forces.
Particle accelerators have played a crucial role in testing and refining these theories. From the early cyclotrons of the 1930s to the powerful synchrotrons of today, these machines have allowed scientists to recreate conditions similar to those present shortly after the Big Bang. The Large Hadron Collider (LHC) at CERN stands as the most advanced facility of its kind, enabling high-energy collisions that reveal new particles and interactions.
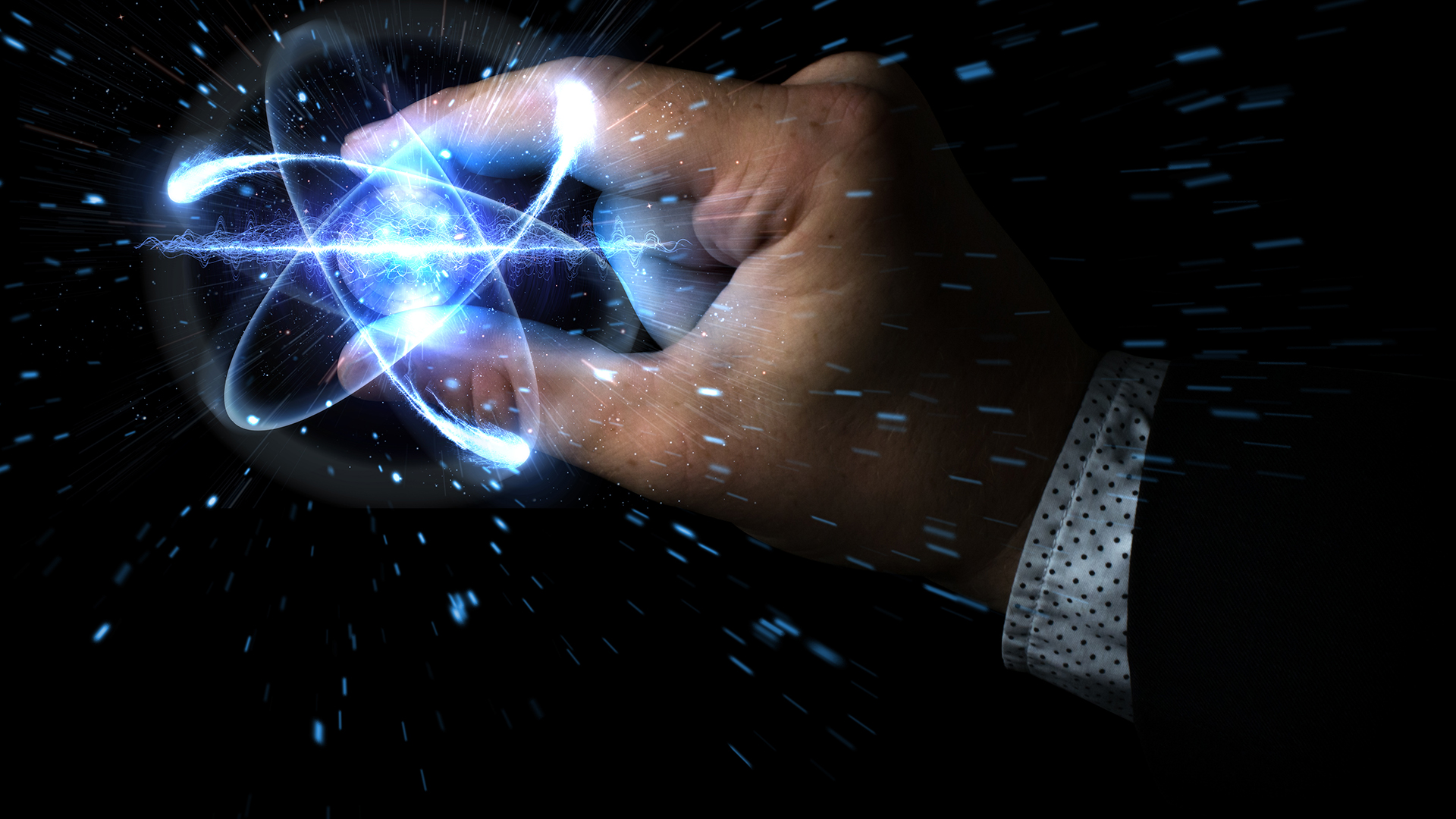
The Impact of the Large Hadron Collider
The LHC has been instrumental in expanding our knowledge of the universe. Its most famous achievement came in 2012 with the discovery of the Higgs boson, a particle that confirms the existence of the Higgs field, which gives mass to elementary particles. This breakthrough completed the Standard Model but also opened new avenues for research.
Beyond the Higgs boson, the LHC has been used to study rare particle decays, test theories beyond the Standard Model, and search for new particles that could hint at the existence of supersymmetry or extra dimensions. However, despite its successes, many fundamental mysteries remain unresolved.
The Limitations of Current Technologies
While the LHC has provided unprecedented insights, it is not without limitations. The accelerator operates at extreme energy levels, yet some phenomena require even higher energies to become observable. The search for new physics is constrained by technological and financial factors, as building larger accelerators presents immense engineering and economic challenges.
Data analysis also represents a bottleneck. The LHC generates enormous amounts of data, requiring sophisticated algorithms and artificial intelligence to identify meaningful patterns. Even with distributed computing projects assisting in simulations and calculations, processing and interpreting this data remain complex tasks.
Future Accelerators and Experimental Approaches
To overcome current limitations, physicists are exploring new accelerator technologies. Several proposals have emerged, including:
The Future Circular Collider (FCC)
The FCC is a proposed next-generation accelerator that would dwarf the LHC in scale and energy capacity. With a circumference of 100 kilometers, it would operate at collision energies nearly four times higher than those of the LHC. This increase in energy could allow for direct observations of physics beyond the Standard Model, potentially uncovering new particles and interactions.
Plasma Wakefield Acceleration
An alternative approach to traditional accelerators involves plasma wakefield acceleration, which uses high-energy plasma waves to accelerate particles over shorter distances. This method could significantly reduce the size and cost of future accelerators while still achieving high energies. While still in experimental stages, this technology has the potential to revolutionize high-energy physics research.
Muon Colliders
Muon colliders present another intriguing possibility. Unlike protons, muons are fundamental particles, meaning they produce cleaner collision data. Additionally, muons can reach higher energies within a smaller accelerator footprint. However, due to their short lifespan, creating and stabilizing a beam of muons remains a significant challenge.
The Role of Artificial Intelligence in Particle Physics
Advancements in artificial intelligence (AI) are increasingly influencing the field of particle physics. AI and machine learning algorithms assist in analyzing collision data, optimizing detector performance, and identifying new patterns in large datasets. By improving the efficiency of simulations and predictions, AI accelerates the pace of discovery and enhances our ability to test theoretical models.
One promising application of AI is in anomaly detection. By identifying unexpected deviations in experimental data, AI-driven systems can flag potential new physics events that might otherwise go unnoticed. Additionally, machine learning aids in reconstructing particle tracks, allowing for more precise measurements of fundamental interactions.
The Intersection of Particle Physics and Cosmology
The discoveries made in high-energy physics laboratories have profound implications for our understanding of the cosmos. Many questions in cosmology, such as the nature of dark matter and dark energy, require insights from particle physics experiments.
Dark Matter Detection
Despite overwhelming evidence for the existence of dark matter, its exact nature remains unknown. The LHC and future accelerators aim to produce dark matter candidates, such as weakly interacting massive particles (WIMPs) or axions, in controlled experiments. Detecting these elusive particles would revolutionize our understanding of the universe’s composition.
The Early Universe and Cosmic Inflation
Particle physics experiments also help probe conditions that existed fractions of a second after the Big Bang. Understanding high-energy interactions allows scientists to test inflationary models and explore the symmetries that governed the universe’s earliest moments. By recreating these conditions, accelerators provide a window into the fundamental laws that shaped the cosmos.
International Collaboration in High-Energy Physics
Given the complexity and cost of modern experiments, international collaboration is essential for progress in particle physics. Organizations like CERN, Fermilab, and KEK bring together scientists from around the world to share resources and expertise. The exchange of ideas and data fosters innovation and ensures that discoveries are accessible to the global scientific community.
Additionally, collaborations between high-energy physics and other fields, such as quantum computing and material science, lead to technological advancements with applications beyond fundamental research. The development of superconducting magnets, advanced detectors, and high-performance computing systems benefits industries ranging from medicine to aerospace.
The Ethical and Philosophical Implications of Particle Physics
Beyond the technical challenges, high-energy physics raises ethical and philosophical questions. As scientists push the boundaries of knowledge, concerns arise about the potential risks associated with high-energy experiments. While extensive safety measures are in place, discussions about the ethical responsibilities of researchers remain relevant.
Furthermore, particle physics challenges our perception of reality. Concepts such as quantum entanglement, extra dimensions, and the multiverse question the very nature of existence. These discoveries not only advance science but also inspire deeper philosophical inquiries about the universe and our place within it.
The Road Ahead for Particle Physics
As research in high-energy physics progresses, the coming decades promise new discoveries that could redefine our understanding of the universe. While the challenges of cost, technology, and data analysis remain significant, the potential rewards far outweigh the difficulties. Whether through next-generation accelerators, AI-driven analyses, or novel experimental techniques, the pursuit of knowledge continues to drive innovation.
With each breakthrough, humanity moves closer to answering some of the most profound questions about the nature of matter, energy, and the forces that govern the cosmos. The future of particle physics is not just about exploring the unknown—it is about expanding the very limits of human understanding.